Scintillator-based in vivo dosimetry during pulsed dose rate brachytherapy for cervical cancer
OC-0448
Abstract
Scintillator-based in vivo dosimetry during pulsed dose rate brachytherapy for cervical cancer
Authors: Peter Georgi1, Søren K. Nielsen2, Anders T. Hansen2, Steffen B. Hokland2, Harald Spejlborg2, Susanne Rylander3, Lars U. Fokdal2, Jacob Lindegaard2, Primoz Petric4, Kari Tanderup2, Jacob G. Johansen2
1Aarhus University, Department of Clinical Medicine, Aarhus, Denmark; 2Aarhus University Hospital, Department of Oncology, Aarhus, Denmark; 3Aalborg University Hospital, Department of Medical Physics, Aalborg, Denmark; 4Zürich University Hospital, Department of Radiation Oncology, Zürich, Switzerland
Show Affiliations
Hide Affiliations
Purpose or Objective
To investigate inter-pulse and -dwell dose variation during pulsed dose
rate (PDR) brachytherapy (BT) for cervical cancer via in vivo dosimetry (IVD) with a scintillation-based detector and utilize time-resolved data to increase IVD reliability.
Material and Methods
At our department IVD with a scintillator-based
detector measuring the dose rate every 50ms is routinely performed during PDR
BT for cervical cancer. In this study the patients received two fractions of 17.5 Gy for tandem ring (TR) treatments and 15 Gy for multi-channel vaginal cylinder (MVC) treatments each divided into 20 hourly pulses. Treatment planning was based on
MRI. Before treatment, the scintillator was placed in a separate channel. The dosimeter position and corresponding expected dose
recording were determined for each plan.
Retrospectively, measured and expected dose were compared for each fraction. Furthermore, the pulse-to-pulse
variations of the dose deviations were investigated to study the implant
stability. A methodology was applied for correcting for uncertainties in detector
position and evaluated in 15 treatments with MVC.
The methodology involved estimation of positional shifts of the dosimeter along
the MVC’s longitudinal axis based on a least square fit of the TG43-calculated
dose rates to the measured ones for the MVC's central channel. Finally, a comparison
of the measured and expected dose rates were performed for each dwell position
in a single treatment. The comparison was done both before and after applying
the positional correction of the detector.
Results
Data from 79 PDR BT fractions was analysed; 33 MVC and 46 TR. The mean±1SD of the deviation between the total measured and expected
dose were -1.1±1.7 Gy (-9±13%) for TR and 0.0±0.5 Gy (0±4%) for MVC. For the
first pulse the dose deviation was -0.4±3% for TR and -0.1±0.6% for MVC, with an
increase in variation (1SD) of up to ±18% and ±12% respectively for subsequent pulses, fig. 1.
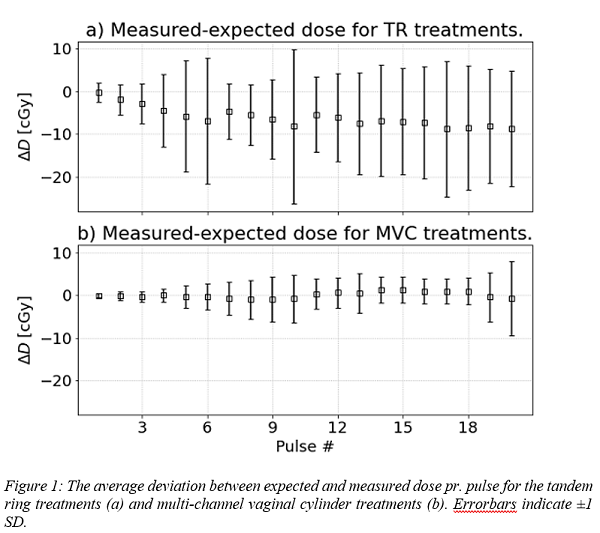
15 MVC treatments showed an average
positional dosimeter correction of 0.5±9 mm. Five of these plans exhibited
deviations of more than 10% between measured and expected dose in one or more
pulses. The mean deviation between measured and expected dose rate pr.
dwell-position in the non-central MVC channels dropped from 22±95% to 3.4±7.9%
after applying the correction for detector position, fig. 2.
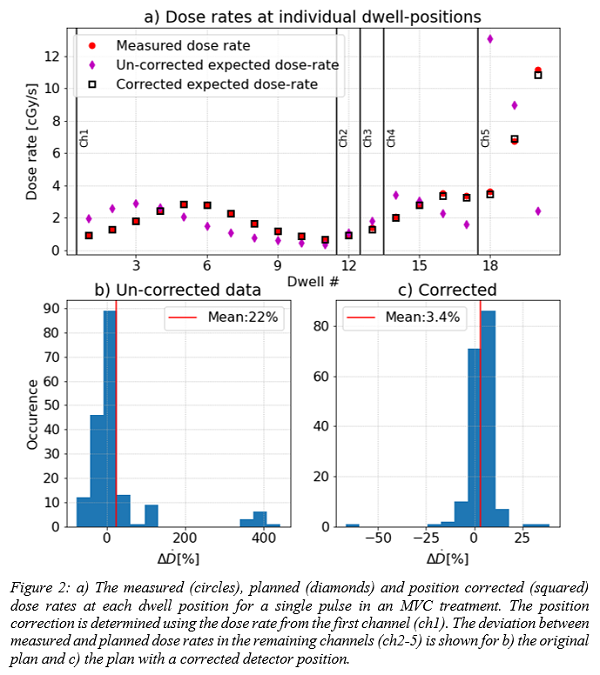
Conclusion
Real-time dosimetry during PDR BT treatments has been performed in a
large cohort, showing good agreement with the expected dose for the MVC. The
larger deviation seen with TR is expected to originate from positional offsets of
the detector. The measured dose rates enabled a more thorough investigation of
the treatment, including determining positional offsets in the detector
position. Correcting for detector position based on the irradiation of the
first source channel increased the reliability of the subsequent dose rate
comparison. The next step is to test this method in the full cohort including
TR+needle treatments.