Need for robust tests to better estimate the different biological parameters involved in tumour response to different radiotherapy protocols - PDF Version
The last 30 years have seen a strong evolution of clinical radiotherapy practices. Until recently, clinicians almost exclusively applied fractionated protocols of 2Gy, administered daily, for 25 to 30 days. Recent developments in physical dosimetry and imaging have enabled the emergence of new radiotherapy treatments (e.g., stereotactic, intensity-modulated, proton, or flash therapies), which allow for the delivery of higher and more precise doses to the tumour than was previously possible, and with reduced toxicity to normal tissue. Radiotherapy can also be combined with the delivery of targeted pharmacological drugs to enhance radiosensitisation of tumour cells. Thus, medical strategies have changed radically and clinicians must define more effective protocols than before, based on multiple physical and oncological parameters that include cumulative dose, number of fractions and time between fractions, or genetic grade of the tumour. However, protocol selection is based primarily on empirical approaches in clinical trials and may be supported by experimental designs.
To assist clinicians in protocol selection, standardised radiobiological tests have been developed. For example, clonogenic survival tests that measure the ability of a tumour cell to survive, divide, and then form a multicellular clone have been used for more than 50 years. The dose-dependent decrease in the number of clones is modelled by a linear-quadratic equation (LQ model) that is widely used to assess and compare the sensitivity of tumour cells in vitro and subsequently to infer the effectiveness of a radiotherapy dose for clinical purposes. However, this robust assay has conceptual limitations because radiomodulated biological events are not observed and quantified directly. Increased rates of cell death, delayed or permanent cell-cycle arrest, abnormal cell divisions or genomic transformations, which are cellular and genetic events that explain tumour radiosensitivity, are not captured in clonogenic survival. This is unfortunate, because it seems logical that these different events in the radiobiological response are induced differently depending on the dose, the quality of the radiation or the dose rate. Detailed knowledge of these events according to the properties of the radiation would enable us to improve our choice of the appropriate radiosensitising drug.
Specific biochemical and immunological assays that employ western blotting, RNA sequencing, fluorescence-activated cell sorting analysis or fluorescent microscopy already enable us to measure these events, but they give a frozen picture of the radiobiological response that occurs at specific time points after irradiation and miss the whole kinetics of these events after irradiation. For example, some events such as cell death occur more rapidly as the dose is increased. Repetition of assays at several time points to obtain the full dynamics of the tumour-cell response to radiotherapy is time-consuming and expensive.
A last point of importance is the singular response of each cell to ionising radiation. Genetic and cellular heterogeneity in the tumour-cell population promotes tumour aggression and resistance to treatment. Furthermore, the stochastic DNA damage in the 23 chromosome pairs that is induced by ionising radiation can lead to different radiobiological behaviours depending on the type of DNA damage and the cells’ repair capacity. In addition, the position in the cell-cycle phase at which the radiation is applied also determines the relative response to radiation, with cells being more radiosensitive in the G2-M phase and radioresistant in the G1 and late S phases.
Thus, there are various bottlenecks in the current use of cellular assays to assess radiobiological response. These bottlenecks limit the biological input of these assays and their potential transfer to clinical trials. This study is based on the assumption that the next generation of radiobiological assays must include the synthesis of high-throughput dynamic biological data at a multi-scale, cellular level (from a single cell to the whole population), so that their use will improve knowledge of tumour radiobiology and lead to multi-parameter models that are based on dose escalation or genetic or pharmacological perturbations.
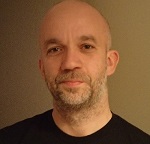
François Paris
Cancer Research Center CRCI²NA
Inserm
Nantes University
Nantes, France