Latest news on biology from the FLASH world - PDF Version
FLASH (ultra-high dose rate radiotherapy) is attracting a lot of attention in the radiotherapy community. The delivery of radiation at ultra-high dose rates has been shown to lead to sparing of normal tissue while the tumour response seems to be the same as that observed during use of conventional dose rates. The recent FLASH radiotherapy and particle therapy (FRPT) conference (https://frpt-conference.org/), which should have been held in Vienna, Austria, but was converted into a very successful online meeting, demonstrated how much work is currently going on in FLASH research. FLASH research is a multidisciplinary field, in which physicists, clinicians and biologists all have much interest. The biology corner is using this opportunity to highlight some of the recent FLASH biology publications – a kind of FLASH-highlight.
Two very good reviews on the radiobiology of FLASH that were published in 2021 offer an overview of the current data [1,2]. The paper from Kacem et al. (Understanding the FLASH effect to unravel the potential of ultra-high dose rate irradiation) [1] reviewed the field with in-depth discussions on the mechanisms that may lie behind the FLASH effect [1]. The review paper from Friedl et al. (Radiobiology of the FLASH effect) [2] contains a very thorough overview of the different models and end-points that are used in FLASH studies, and the underlying biology. The paper from Friedl et al. is published in a special issue of Medical Physics on FLASH.
One of the questions currently taxing researchers in FLASH is how fractionation influences the FLASH effect. A preclinical study that tested different fractionation schemes was performed by Montay-Gruel and colleagues in 2021 [3]. In this study, tumour response and the sparing of normal tissue were investigated simultaneously in the same animals, which were irradiated with either electron FLASH or conventional radiotherapy (CONV-RT). The end-points were tumour growth, which was measured by use of a well-characterised murine orthotopic glioblastoma model, and neurocognitive side-effects, which were evaluated through cognitive testing of brain-irradiated mice. The authors demonstrated that 10Gy in 1 fraction, 2x7Gy and 3x10Gy doses led to delayed tumour growth comparable with that achieved through use of CONV-RT alongside neurocognitive sparing. On the other hand, doses of 14Gy in 1fraction and 3.5x4Gy also delayed tumour growth but induced neurocognitive deficiencies that were similar to those caused by CONV-RT. The authors also analysed the temporal dosimetry characteristics of the published experimental data for the FLASH effect in vivo. Taken together, the data in this paper reveal that a FLASH effect can be achieved with application of a fractionated scheme, but that a lot of work remains to be performed to understand all the different factors that apparently influence FLASH, such as instantaneous dose rate, time structure, radiation fraction size, numbers of fractions and total dose.
Most FLASH experimental data are based on electron irradiation; however, studies of proton FLASH are emerging. A review in the previously mentioned special issue of Medical Physics, published last year, focused on the current status of proton FLASH [4]. Diffenderfer and colleagues discussed current studies on proton FLASH from both the physics and the radiobiology viewpoints. Two papers that were also published in 2021 carried experimental biology data that came from proton FLASH studies [5,6]. Velalopoulou and colleagues compared the effects on mouse legs of doses of FLASH (69-124Gy/s) and of CONV-RT (0.39-0.65Gy/s) through use of a doublet scattered proton beam [5]. The effects were studied in several ways: one was through transcriptome analysis of skin that was taken from the irradiated mouse legs five days after irradiation doses of 30Gy. The analysis revealed differential gene expression between the two modalities; pathways that were associated with apoptosis, apoptotic signalling and keratin signalling were upregulated uniquely by CONV-RT. This confirmed observations of reduced skin damage and soft tissue reactions in the FLASH group compared with the CONV-RT group when both groups were irradiated with the same dosage (30Gy). Interestingly, application of FLASH induced upregulation of pathways that were related to tissue and vascular repair in the skin, including anatomical structure morphogenesis, blood vessel morphogenesis, and vascular development [5].
Cunningham and colleagues demonstrated a FLASH effect with application of pencil-beam scanning proton FLASH using a clinical gantry in a study of radiation effects on mice legs [6]. In this case, 35Gy was delivered either at a rate of 1Gy/s (CONV-RT) or 57Gy/s (average dose rate) in a 25mmx23mm field. The effect on skin toxicity, leg contraction, and on levels of tumour growth factor β1 (TGF-β1) and other cytokines in the plasma and the skin, was assessed. The researchers found that the effect on these parameters in the FLASH group was lower than that observed in the CONV-RT group. The effect of an even higher dose rate, 115Gy/s, was unchanged from that at the dose rate of 57Gy/s on leg contraction and TGF-β1 levels. In the same set-up, the growth rate of tumours in two mouse oral carcinoma cell lines, MOC1 and MOC2, following dosage of 15Gy was no different between tumours that were irradiated at a conventional dose rate or at the high rate of 57Gy/s [6].
The FLASH effect remains to be elucidated at the mechanistic level, and there are more current hypotheses. In more of these theories, oxygen plays a role. This suggestion is supported by a recent report that investigated application of FLASH and CONV-RT in clonogenic survival assays. The results of this study indicated the importance of the oxygen level [7]. Oxygen depletion, which leads to transient hypoxia, has been proposed as a mechanism of the FLASH effect, and more papers have been published on this subject. One of these is a paper from Rothwell and colleagues, in which the authors present the development of a model of oxygen consumption and reaction in cells following FLASH irradiation [8]. Several research groups have used various methods to investigate whether oxygen consumption is a possible explanation for the FLASH effect. These methods include Monte Carlo track structure analysis, calculations and measurements of oxygen consumption in water due to radiolysis [9-11]. In one study, by Cao et al., the oxygen depletion at FLASH dose rates compared with CONV-RT was quantified in vitro and in vivo through use of a phosphorescence quenching method and a water-soluble molecular probe [12]. The data from this study suggest that oxygen depletion to radiologically relevant levels of hypoxia is unlikely to occur in tissue that undergoes FLASH irradiation.
It was clear at the FRPT meeting that various groups were currently undertaking considerable work and that a large amount of data would emerge soon. Together, these data may supply the necessary information from the biology viewpoint to solve the puzzle of which parameters are influencing the effect of FLASH and to enable the translation of FLASH into clinical practice.
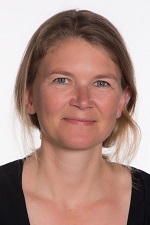
Brita Singers Sørensen
Professor at the Danish Centre for Particle Therapy and
at Department of Experimental Clinical Oncology,
Aarhus University Hospital
Aarhus, Denmark
References:
- Kacem H, Almeida A, Cherbuin N, Vozenin M-C. Understanding the FLASH effect to unravel the potential of ultra-high dose rate irradiation. Int J Radiat Biol 2021, DOI: 10.1080/09553002.2021.2004328
- Friedl AA, Prise KM, Butterworth KT, Montay-Gruel P, Favaudon V. Radiobiology of the FLASH effect. Med Phys 2021, DOI: 10.1002/mp.15184
- Montay-Gruel P, Acharya MM, Jorge PG, Petit B, Petridis IG, Fuchs P, et al. Hypofractionated FLASH-RT as an effective treatment against glioblastoma that reduces neurocognitive side effects in mice. Clin Cancer Res 2021;27:775-84
- Diffenderfer ES, Sørensen BS, Mazal A, Carlson DJ. The current status of preclinical proton FLASH radiation and future directions. Med Phys 2021, DOI: 10.1002/mp.15276
- Velalopoulou A, Karagounis I V., Cramer GM, Kim MM, Skoufos G, Goia D, et al. FLASH Proton Radiotherapy Spares Normal Epithelial and Mesenchymal Tissues While Preserving Sarcoma Response. Cancer Res 2021;81:4808-21.
- Cunningham S, Mccauley S, Vairamani K, Speth J, Girdhani S, Abel E, et al. FLASH Proton Pencil Beam Scanning Irradiation Minimizes Radiation-Induced Leg Contracture and Skin Toxicity in Mice. Cancers 2021;13.
- Adrian G, Konradsson E, Lempart M, Bäck S, Ceberg C, Petersson K. The FLASH effect depends on oxygen concentration. Br J Radiol 2020;93.
- Rothwell BC, Kirkby NF, Merchant MJ, Chadwick AL, Lowe M, Mackay RI, et al. Determining the parameter space for effective oxygen depletion for FLASH radiation therapy. Phys Med Biol 2021;66:055020.
- Boscolo D, Scifoni E, Durante M, Krämer M, Fuss MC. May oxygen depletion explain the FLASH effect? A chemical track structure analysis. Radiother Oncol 2021;162:68-75.
- Jansen J, Knoll J, Beyreuther E, Pawelke J, Skuza R, Hanley R, et al. Does FLASH deplete oxygen? Experimental evaluation for photons, protons, and carbon ions. Med Phys 2021;48:3982-90.
- Favaudon V, Labarbe R, Limoli CL. Model studies of the role of oxygen in the FLASH effect. Med Phys 2021, DOI: 10.1002/mp.15129
- Cao X, Zhang R, Esipova T V, Allu SR, Ashraf R, Rahman M, et al. Quantification of oxygen depletion during FLASH irradiation in vitro and in vivo. Int J Radiat Oncol Biol Phys 2021;111:240-248.